Abstract
Carbon dioxide (CO2) is one of the main greenhouse gases (GHGs) responsible for the current trends in climate change. The fifth Intergovernmental Panel on Climate Change (IPCC) report suggests that in order to have a greater than 50% chance of limiting global warming to 1.5°C by 2050, we will need to remove 5–10 billion tons of CO2 from the atmosphere annually. Moreover, the Paris Agreement’s pledged pathways to net-zero carbon emissions depend on both CO2 removal and reduction. To curb the ever-increasing CO2 concentrations, various technologies have been proposed globally including Carbon Capture and Storage (CCS). CCS works in three steps: (i) carbon capture, (ii) compression and transportation, and (iii) injection into underground rock formations for permanent storage. Compared to sedimentary rocks, basalts have demonstrated a higher potential for CCS. The Kenya Rift has abundant basalt formations that can be suitable for permanently storing CO2. Despite CCS being a promising strategy to mitigate CO2 emissions, its application in geologically complex areas like the Kenya Rift requires a comprehensive understanding of associated risks. This article evaluates potential risks of CCS along the Kenya Rift, focusing on geological, environmental and socio-economic factors. Kenya’s commitment to reducing carbon emissions, as outlined in its Nationally Determined Contributions (NDCs), highlights the need for innovative solutions like CCS. Understanding the risks will ensure that CCS contributes positively to Kenya’s climate goals without unintended consequences.
Keywords: Basalts, Carbon Capture and Storage, Climate Change, CO2 emissions, Geologic Carbon Sequestration, Kenya Rift
Introduction
In 2023, global carbon dioxide emissions from cement and fossil fuels surged by 1.1%, reaching a new all-time high of 36.8 GtCO2 (2023 Global Carbon Budget report). The oceans and terrestrial biosphere are responsible for absorbing half of these emissions naturally (Okoko and Olaka, 2021). The remainder intensifies Earth’s natural greenhouse gas effect and exacerbates climate change. Mineral carbonation, deep ocean storage and underground geological storage are potential strategies for sequestering the remaining CO2. The most established CCS is done in deep sedimentary layers, where at least 43 CCS projects have been conducted in America, Europe and some in China (Okoko and Olaka, 2021). One limitation of CCS in sedimentary layers is that the calcium and magnesium-rich silicate minerals required for mineral carbonation are absent in sedimentary rocks making the reactions very slow and can take thousands of years (White et al., 2005). This shortcoming has led to the recent shift to mafic rocks such as basalts for CCS projects.
Carbonate mineralization can take less than two years in basalt rocks (Matter et al., 2016). Divalent cations found in basalts, such as Ca2+, Mg2+ and Fe2+, enhance the reaction with CO2 to produce stable carbonate minerals, including zeolite, siderite, calcite and magnesite (Pokrovsky et al., 2009). Various basaltic regions have been assessed for CCS viability. These projects include Wallula Basalt project on the Columbia River Flood basalts, deep-sea basalts from the Juan de Fuca Ridge and the Iceland CarbFix project. The Kenya Rift, part of the East African Rift System, is characterized by extensive basalt provinces, active tectonics, geothermal activity and complex subsurface geology. These factors present unique challenges and opportunities for CCS implementation.
Despite Africa’s low CO2 emissions in comparison to industrialized countries, the continent’s aspirations for rapid industrialization is likely to result in increased anthropogenic CO2 emissions in the next decades (Bamisile et al., 2021). For instance, CO2 emissions in Kenya were 21,527,440 tons in 2022, equivalent to 0.40 tons per person based on a population of 54,252,461 in 2022 (Fig. 1). Anthropogenic sources of CO2 emissions near the Kenya rift include the burning of fossil fuels, the manufacturing of cement and the production of geothermal energy (Okoko and Olaka, 2021).
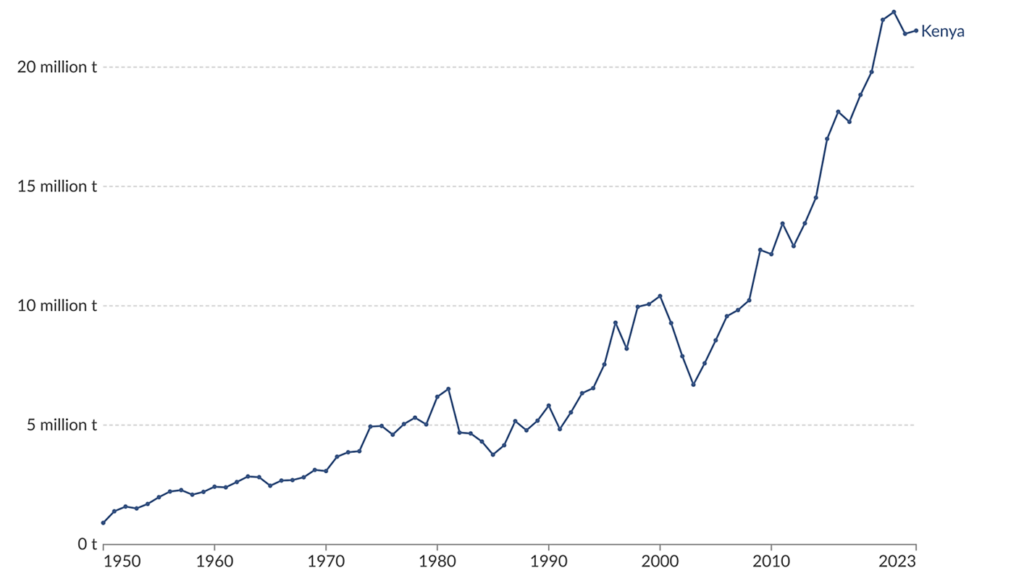
Fig. 1. Graph showing the annual CO2 emission from 1950 -2023 in Kenya (data source, Ritchie et al., 2020).
Geological Setting of the Kenya Rift
About 900 km long and spanning latitudes 5° N to 3° S, the Kenya rift section is made up of three main asymmetric rift basins: the Northern, Central and Southern Kenya Rifts (Okoko and Olaka, 2021), each of which varies in width from 50 to 70 km. The Central Kenya rift is narrow, whereas the northern and southern portions are wider.
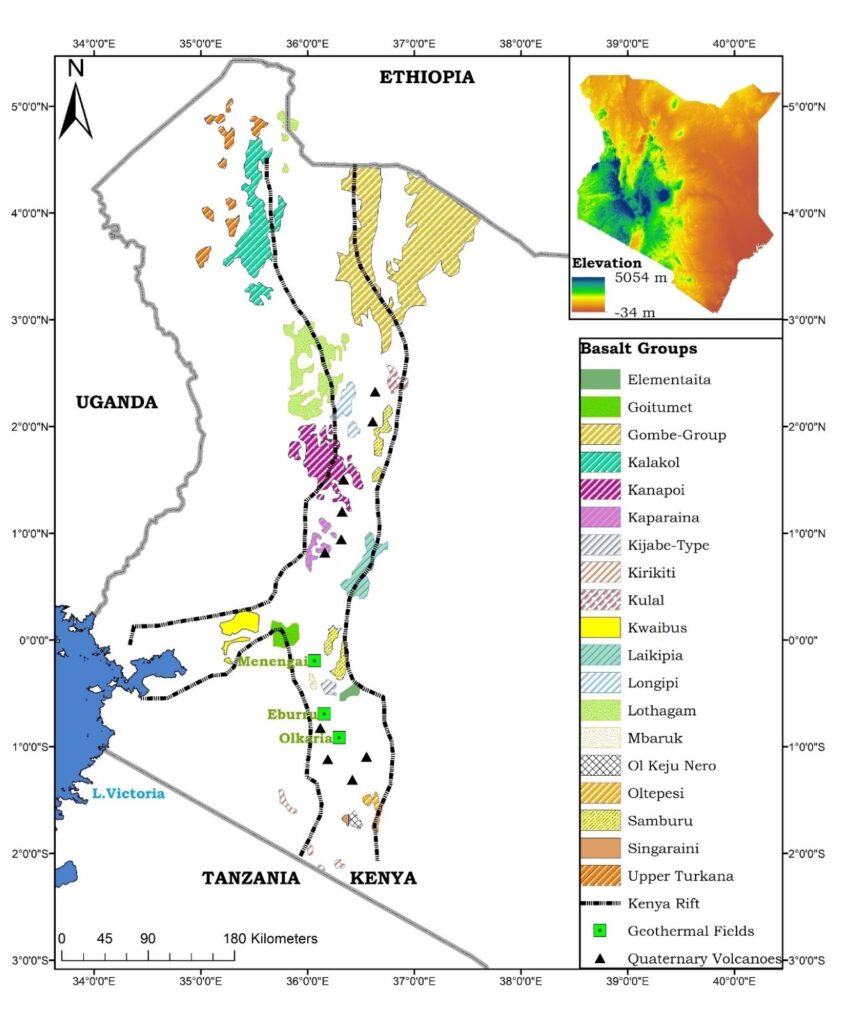
Fig.2. Regional map of the Kenya Rift System overlain by basalt groups, geothermal fields and quaternary volcanoes.
Basalts within the Northern Kenya Rift (NKR) are larger than the Central (CKR) and Southern (SKR) basalts (Fig. 2). NKR is comprised of seven distinct basalt groups: Upper Turkana, Kalakol, Gombe Group, Samburu, Logipi, Lothagam and Kulal basalts. CKR has six basalt groups: Mbaruk, Kaparaina, Goitumet, Kijabe-type, Elementaita and Kwaibus basalts. SKR basalts include Ol Tepesi, Kirikiti, Singaraini, Ol Keju Nero basalts.
Why the Kenya Rift is an Ideal Candidate for CCS Projects
Physical Attributes of the Basalts
Along the Kenya Rift, basalts are often found in thick, extensive layers resulting from multiple episodes of volcanic activity. This substantial thickness enhances their ability to store large volumes of CO₂. The Basalts also have significant primary porosity, which refers to the void spaces within the rock created during lava cooling. This porosity allows the injected CO₂ to infiltrate and occupy available pore spaces.
Tectonic activity in the Kenya Rift has introduced fractures into the basalts, further enhancing their permeability. These fractures provide pathways for CO₂ injection and increase the overall storage capacity. The porous structure of basalts facilitates the interaction of CO₂ with minerals like calcium, magnesium, and iron within the rock. These reactions can permanently convert CO₂ into stable carbonate minerals, effectively locking it away.
Table 1. Estimated thickness, area, volume and porosity of basalts along with the Kenya Rift System (data from Okoko and Olaka, 2021)
Basalt Groups | Thickness (km) | Area (km²) | Volume (km³) | Porosity |
Elementaita | ? | 231.2 | ? | Good |
Goitumet | 0.2 | 527.1 | 118.6 | Moderate |
Gombe-Group | 0.2 | 11342.2 | 2268.5 | Moderate |
Kalakol | 0.8 | 3696.2 | 2,901.5 | Moderate |
Kanapoi | ? | 2443.7 | ? | Moderate |
Kaparaina | 0.6 | 443.8 | 266.3 | Moderate |
Kijabe-Type | 0.05 | 311.9 | 14.2 | Good |
Kirikiti | 0.3 | 242.8 | 73.6 | Moderate |
Kulal | ? | 339.7 | ? | Moderate |
Kwaibus | 0.2 | 772.7 | 173.9 | Moderate |
Laikipia | 0.6 | 1171.7 | 710.8 | Moderate |
Longipi | 0.3 | 571 | 171.3 | Moderate |
Lothagam | 0.1 | 2946.9 | 147.4 | Moderate |
Mbaruk | 0.03 | 49.6 | 1.5 | Good |
Ol Keju Nero | 0.1 | 122.6 | 1.1 | Good |
Elgeyo | 0.9 | 404.6 | 364.1 | Moderate |
Samburu | 1.0 | 1011.6 | 1011.6 | Moderate |
Singaraini | ? | 27.9 | ? | Moderate |
Upper Turkana | 1.0 | 808.3 | 808.3 | Moderate |
Availability of Water
The Kenya Rift Floor, with its significant groundwater reserves and Rift Valley lakes, provides a reliable water supply, making the region favorable for CCS implementation. The substantial aquifers replenished by rainfall and inflows from surrounding highlands, provide the water needed for CO₂ dissolution prior to injection. In deep injection scenarios, water plays a role in maintaining reservoir pressure, ensuring efficient CO₂ storage. The lakes Turkana, Logipi, Baringo, Bogoria, Nakuru, Elementaita, Naivasha, Magadi, and Ol Bolossat are situated along the Kenya Rift. These lakes could serve as supplementary water resources for CCS operations.
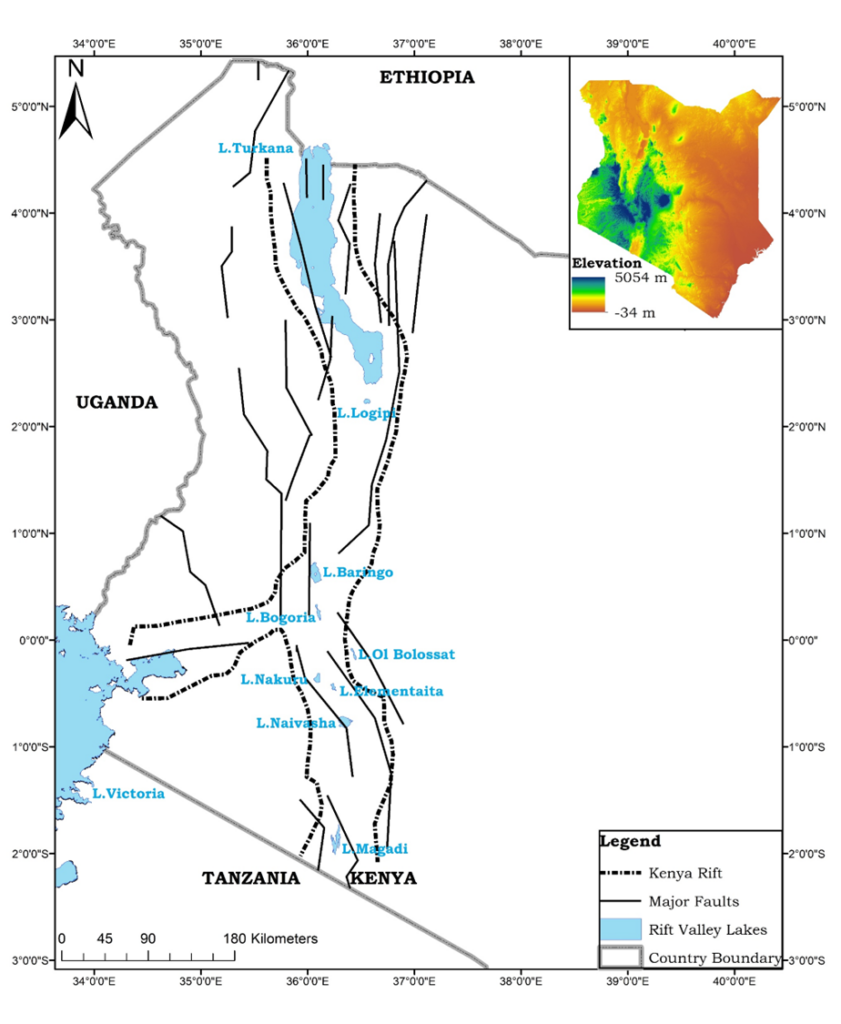
Fig.3. Regional map of the Kenya Rift System overlain by rift valley lakes and major faults
Subsurface Temperature
Low temperatures between 20° and 40°C and high temperatures between 40° and 150°C can both be used for CO2 sequestration. The best sequestration occurs at high temperatures because mineralization occurs more quickly and less water is needed to absorb the same quantity of CO2 at low temperatures (Okoko and Olaka, 2021). The temperatures in the Kenyan rift are often higher than those in other CCS project regions, such Juan de Fuca, where they vary between 62 and 64°C at 600 meters below the surface (Goldberg et al., 2008). This implies that Kenya rift’s subsurface temperatures could be perfect for CCS (Fig.4, Table 2).
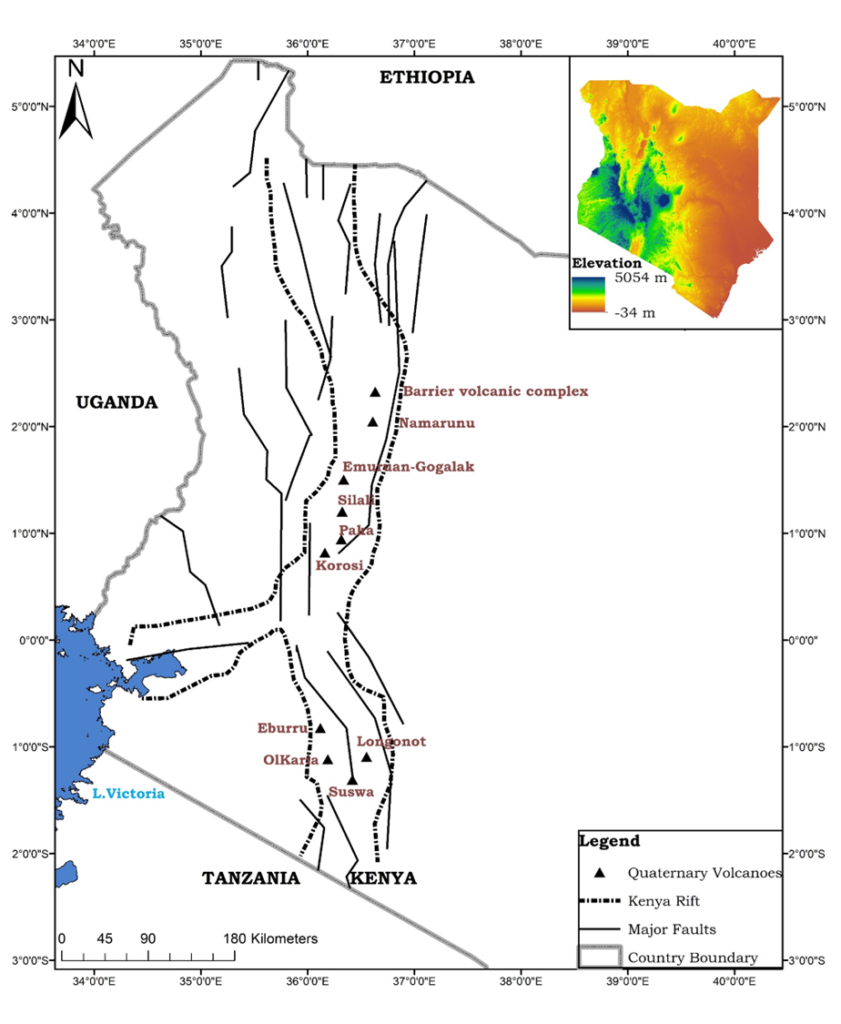
Fig.4. Regional map of the Kenya Rift System overlain by quaternary volcanoes and major faults
Table 2. Areas of high ground temperatures within the Kenya rift (data from Okoko and Olaka, 2021)
Locality | Subsurface Temperature (°C) |
Barrier Volcanic Complex | 82-96 |
Namarunu | 85-97 |
Emurian-Gogalak | 80-96 |
Silali | 96.8 |
Paka | 97.8 |
Korosi | 80-96 |
Eburru | 80-96 |
Olkaria | 85-97 |
Longonot | 90 |
Suswa |
Proximity to Geothermal Systems
The Kenya Rift is home to some of the most significant geothermal energy resources in Africa, including the well-known Olkaria, Menengai and Eburru geothermal fields (Fig.2). This proximity to geothermal sites provides a unique advantage for CCS projects, creating opportunities for infrastructure sharing, cost reduction and enhanced storage efficiency. The significant levels of CO2 that are already naturally fixed in geothermal systems reflect how well basaltic rocks store energy (Okoko and Olaka, 2021). Moreover, accessibility to geothermal sites minimizes the need to construct extensive pipelines for CO₂ transport, as capture facilities can be located near injection sites.
Kenya’s national energy strategy prioritizes geothermal energy as a key renewable resource. Coupling geothermal with CCS aligns with Kenya’s climate commitments, such as reducing greenhouse gas emissions under the Paris Agreement. Combining geothermal and CCS operations in the same location creates integrated systems that maximize resource use while minimizing environmental footprints.
Potential Risks of CCS Projects in Kenya
Geological Risks
The Kenya Rift presents several geological risks for Carbon Capture and Storage (CCS) projects. While the region offers significant potential for CO₂ sequestration, these risks must be thoroughly assessed and managed to ensure the safety and effectiveness of storage operations.
Induced Seismicity: The Kenya Rift is characterized by frequent seismic activity due to ongoing tectonic forces associated with the rifting process. CO₂ injection can increase reservoir pressure, potentially triggering seismic events in already stressed geological formations. Induced seismicity may: reactivate dormant faults, yield M6 magnitude earthquakes or disrupt nearby infrastructure and ecosystems.
Fault Integrity: The Kenya Rift is crisscrossed by numerous faults and fractures resulting from tectonic stretching. These features can compromise CO₂ storage by acting as conduits for gas migration to the surface or into unwanted geological layers. Increased pressure from CO₂ injection can reactivate faults, further exacerbating the risk of leakage. The likelihood of reactivation depends on factors such as fault orientation, stress regime and proximity to injection sites. Additionally, faulting can breach overlying cap rocks, which are essential for trapping CO₂ within the reservoir. A compromised cap rock significantly reduces storage security.
Reservoir Suitability: The properties of reservoir rocks, such as porosity and permeability, vary significantly across the Kenya Rift due to its complex volcanic and sedimentary history. This variability impacts injection efficiency where heterogeneous reservoirs with irregular permeability can lead to uneven CO₂ distribution, increasing the risk of pressure buildup and localized leakage. Despite basaltic formations being promising for mineralization, not all basalt layers are suitable (Table 1) due to differences in fracture density and mineral composition.
Environmental Risks
These risks include groundwater contamination, Rift Valley lakes inflow and outflow dynamics, and ecosystem disruption.
Groundwater Contamination: CO₂ leakage into groundwater can dissolve in water, forming carbonic acid. This process lowers the pH of the water, leading to acidification of aquifers. Acidified water can dissolve naturally occurring minerals in surrounding rocks, releasing heavy metals (such as lead and arsenic) and other harmful substances into the water. Contaminated groundwater poses health risks to communities relying on aquifers for potable water. Also, groundwater-dependent ecosystems, such as wetlands and springs, may be adversely affected by changes in water chemistry. Acidified groundwater can also dissolve calcite (CaCO₃) and dolomite (CaMg(CO₃)₂), releasing calcium (Ca²⁺) and magnesium (Mg²⁺) ions into the water. This can cause an increase in hardness, hence affecting the use of the groundwater for drinking and industrial processes. Furthermore, acidification may lead to the dissolution of sulfate-bearing minerals such as gypsum (CaSO₄·2H₂O). High sulfate levels can cause a laxative effect in humans and lead to scaling in water distribution systems.
An example is shown below where existing boreholes in the Central Kenya Rift already have elevated Calcium (Ca²⁺) and Sulfate (SO₄²⁻) that are above the recommended World Health Organization (WHO) limits (Fig.5). CO₂ leakage may further deteriorate the groundwater quality and elevate the levels of such parameters.
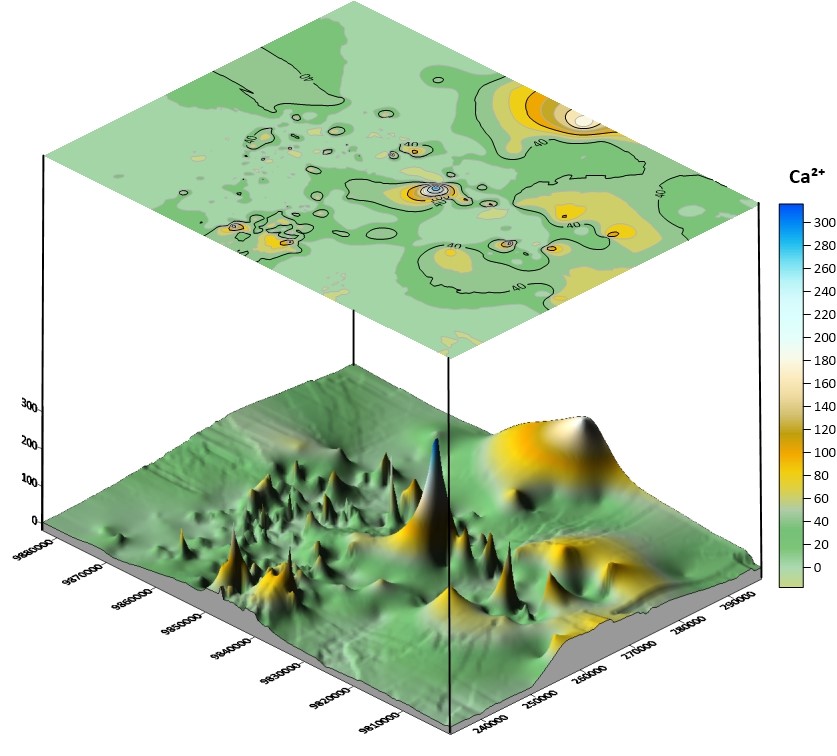
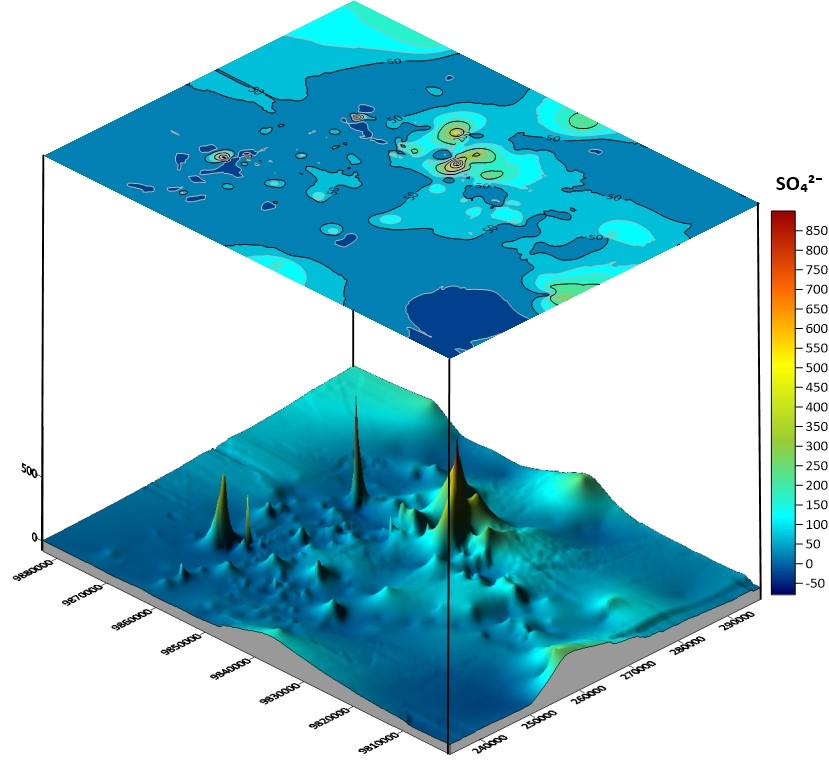
Fig.5. Contour and 3D surface of Ca²⁺ (left) and SO₄²⁻ (right) based on Central Kenya Rift borehole data
Rift Valley lakes inflow and outflow dynamics: Injecting CO₂ into subsurface reservoirs can alter groundwater flow patterns. Since Rift Valley lakes often rely on subterranean aquifers, changes in groundwater movement could affect the lakes’ inflow or outflow dynamics. Rift Valley lakes are biodiversity hotspots. Changes in water chemistry or temperatures due to CO₂ interactions could disrupt aquatic ecosystems, affecting species composition and ecosystem health. CO₂ injection could induce minor seismic events in this tectonically active region. This might destabilize lake shores, especially for expanding lakes with already fragile banks. If induced seismicity increases subsurface permeability, it could lead to additional water seepage into the lakes, exacerbating expansion The Kenyan lakes in the Rift have witnessed significant water level increases. Recent increases in lake areas range from 21% for Lake Naivasha to 123% for Lake Solai (Herrnegger et al., 2021).
Table 3. Changes in the Size of the Affected Lakes (Adapted from Herrnegger et al., 2021)
Lake | 2010 Extent (km²) | 2020 Extent (km²) | Change (2020-2010) | % change |
Turkana | 7947.85 | 8265.07 | 317.22 | 3.99 |
Logipi | 183.46 | 190.93 | 7.47 | 4.07 |
Baringo | 159.49 | 268.06 | 108.57 | 68.07 |
Bogoria | 34.79 | 43.25 | 8.46 | 24.32 |
Nakuru | 45.01 | 68.18 | 23.17 | 51.48 |
Ol Bolossat | 39.81 | 52.16 | 12.35 | 31.02 |
Elementaita | 21.54 | 22.97 | 1.43 | 6.64 |
Naivasha | 154.16 | 193.48 | 39.32 | 25.51 |
Magadi | 148.09 | 153.3 | 5.21 | 3.52 |
One dire situation is Lake Baringo and Lake Bogoria (Fig.6). This is because as the lakes increase in size, the closer they get to each other, and there are fears that the two will combine and become one lake, which would be an ecological disaster as one, Lake Baringo, is a freshwater lake, and the other is an alkaline lake. Originally 20 kilometers apart, the two lakes are now 13 kilometers apart. Interestingly, there is no outlet in each lake to allow excess water to flow out. Associated risks from CO2 injection could exacerbate this expansion.
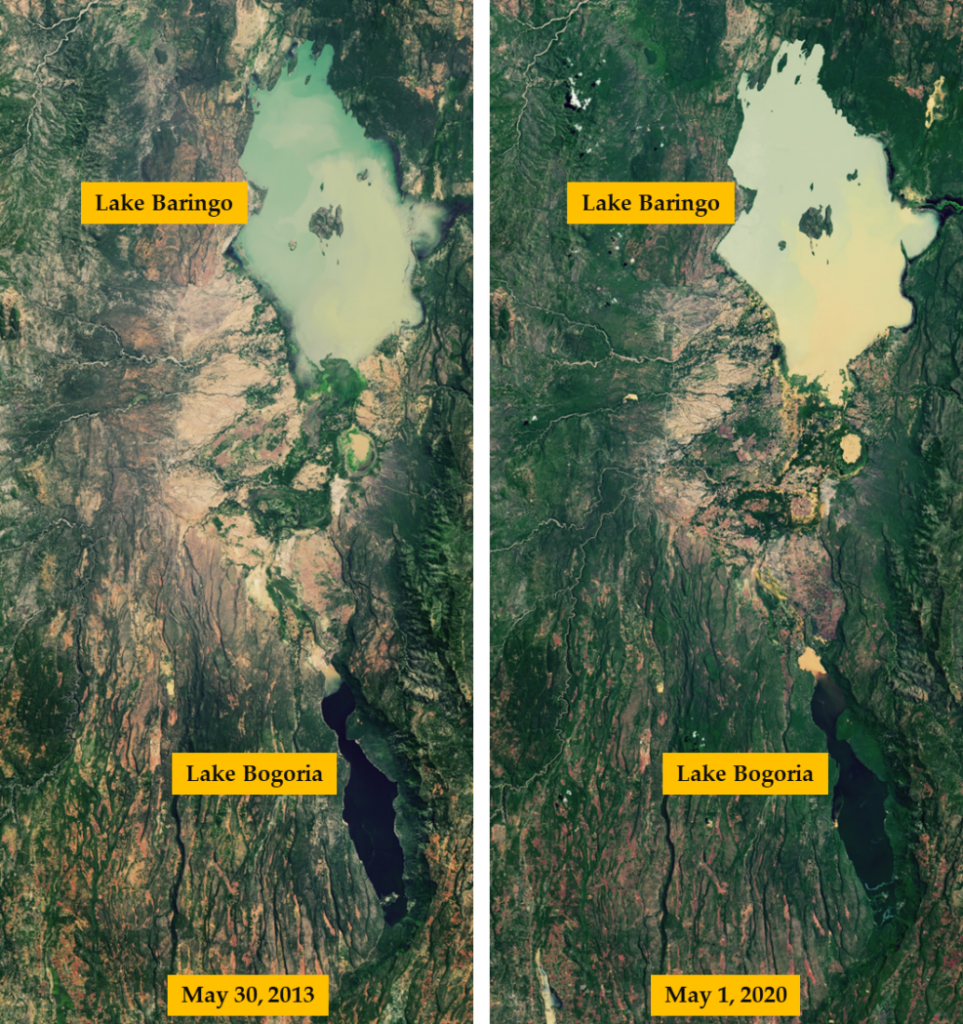
Fig.6. Landsat images showing water levels on Lakes Baringo and Bogoria on May 30, 2013 (left) and May 1, 2020 (right) (Data source: https://earthobservatory.nasa.gov/)
Ecosystem Disruption: The Kenya Rift hosts unique geothermal ecosystems characterized by thermophilic organisms and specialized flora and fauna. CO₂ leakage or changes in subsurface conditions could destabilize these ecosystems. Leakage may alter soil chemistry, groundwater availability or surface temperatures, impacting biodiversity. Endemic species adapted to geothermal conditions could be at risk if their habitats are disrupted by leakage or pressure changes.
Socio-economic Risks
These include land use conflicts, community acceptance and economic viability, all of which must be addressed to ensure successful implementation.
Land Use Conflicts: The Kenya Rift is a multi-use landscape supporting agriculture, pastoralism, human settlements, conservation areas and geothermal energy development. The allocation of land for CCS projects may overlap with these existing uses, creating disputes. Communities, often dependent on land for their livelihoods, may resist land acquisitions or restrictions associated with CCS sites, especially if compensation or alternatives are inadequate.
Community Acceptance: Many communities may be unfamiliar with CCS technology, its purpose, and potential benefits, leading to skepticism. Historical instances of land grabbing, inadequate compensation or lack of public consultation may lead to mistrust in government-led CCS initiatives.
Economic Viability: The size of the project, the location of the CCS facility and the specific characteristics of the CO2 source will all affect the overall cost of a CCS project. These costs include purification to greater than 95% purity, CO2 liquefaction, CO2 transport, CO2 injection, and continuous monitoring (Kelemen et al., 2019). These high upfront costs may deter private sector investment. Unpredictable geological factors, such as reservoir capacity or leakage risks, can create financial risks for investors. Also, the absence of a robust carbon market in Kenya and limited financial incentives for carbon sequestration reduce the economic appeal of CCS projects.
Integrative Risk Management
Following the announcement by the Swiss business Climeworks that it is exploring possibilities for a large-scale facility in the nation, Kenya is poised to become a center for carbon capture and storage. Separately, Project Hummingbird, which is headed by the startup Octavia Carbon, plans to capture 1,000 tonnes of carbon yearly in Kenya. Cella Mineral Storage, Octavia’s partner company, will mineralize the captured carbon and store it for millions of years in the basaltic rocks of the Rift Valley.
In the scenario that this project will be implemented, then successful implementation will require a holistic approach that addresses geological, environmental and socio-economic risks in a coordinated manner. Integrating risk management across these domains ensures safe, sustainable, and socially inclusive CCS operations. Below is a combined strategy to mitigate these risks comprehensively:
- Stakeholder Engagement and Collaboration
- Engage diverse stakeholders, including government agencies, researchers, private sector players, local communities, civil society and environmental organizations, to align interests and foster collaboration.
- Establish clear communication channels to address public concerns about risks such as groundwater contamination, land conflicts and displacement.
- Regional and international collaboration to learn from successful CCS projects in similar geological and socio-economic contexts.
- Advanced Planning and Site Selection
- Use comprehensive geological and geophysical studies to select reservoirs with stable structures, minimal faulting and favorable porosity and permeability.
- Identify and avoid sensitive ecosystems and critical groundwater aquifers during site selection.
- Establish buffer zones around biodiversity hotspots to prevent ecosystem disruption.
- Avoid areas with high land-use conflict potential and prioritize sites with minimal displacement risks.
- Monitoring and Adaptive Management
- Install advanced monitoring systems, such as seismic sensors, groundwater quality probes and surface deformation trackers, to detect early warning signs of seismic activity, CO₂ leakage or subsidence.
- Utilize satellite-based techniques like InSAR (Interferometric Synthetic Aperture Radar) for large-scale monitoring of land deformation.
- Continuously evaluate monitoring data to adapt injection practices and minimize risks.
- Risk Mitigation Strategies
- Control injection pressures and rates to minimize induced seismicity and prevent fault reactivation.
- Use robust well designs and cap rock integrity testing to ensure CO₂ containment.
- Implement geochemical barriers and sealant technologies to protect groundwater from CO₂-induced acidification.
- Design CCS projects as multi-use facilities (such as combining geothermal energy and CCS) to maximize land utility and economic viability.
- Financial and Policy Support
- Offer tax breaks, subsidies and carbon credits to attract private sector investment and offset high initial costs.
- Leverage international climate finance mechanisms, such as the Green Climate Fund (GCF), to support CCS projects.
- Integrate CCS into Kenya’s national climate strategies and align with global frameworks such as the Paris Agreement.
- Promote Public-Private Partnerships (PPPs) to share financial risks and responsibilities, combining public oversight with private sector innovation.
Conclusion
The Kenya Rift presents a unique opportunity for CCS projects, offering favorable geological conditions such as thick basalt formations, adequate porosity, subsurface temperatures and proximity to geothermal energy sites. These features make the region a promising candidate for contributing to global climate change mitigation efforts. However, the successful implementation of CCS in this region requires addressing a range of geological, environmental and socio-economic risks.
Geological challenges demand meticulous site selection, advanced monitoring and controlled injection practices to ensure CO₂ containment and long-term storage security. Environmental risks necessitate robust safeguards to protect water resources, biodiversity and local communities. Socio-economic risks underscore the importance of transparent stakeholder engagement and supportive policy frameworks. Integrating these risk management strategies within a collaborative framework will ensure that CCS projects align with Kenya’s sustainable development goals while maintaining environmental integrity and social inclusivity.
In conclusion, CCS in the Kenya Rift is not merely a technical endeavor but a multidisciplinary challenge requiring synergy between science, policy and community participation. With proactive planning and an integrated approach to risk management, Kenya can harness the potential of CCS to reduce carbon emissions, safeguard natural resources and drive sustainable growth. This study highlights the critical need for continued research, stakeholder collaboration and adaptive strategies to make CCS a viable and transformative solution in the Kenya Rift and beyond.
List of References
Hannah Ritchie, Max Roser and Pablo Rosado (2020) – “CO₂ and Greenhouse Gas Emissions”. Published online at OurWorldinData.org. Retrieved from: https://ourworldindata.org/co2-and-greenhouse-gas-emissions [Online Resource]
Bamisile, O., Obiora, S., Huang, Q., Yimen, N., Idriss, I. A., Cai, D., & Dagbasi, M. (2021). Impact of economic development on CO2 emission in Africa; the role of BEVs and hydrogen production in renewable energy integration. International Journal of Hydrogen Energy, 46(2), 2755-2773. https://doi.org/10.1016/j.ijhydene.2020.10.134
Herrnegger, M., Stecher, G., Schwatke, C., & Olang, L. (2021). Hydroclimatic analysis of rising water levels in the Great rift Valley Lakes of Kenya. Journal of Hydrology: Regional Studies, 36, 100857. https://doi.org/10.1016/j.ejrh.2021.100857
Kelemen, P., Benson, S. M., Pilorgé, H., Psarras, P., & Wilcox, J. (2019). An overview of the status and challenges of CO2 storage in minerals and geological formations. Frontiers in Climate, 1, 482595. http://dx.doi.org/10.3389/fclim.2019.00009
Matter, J. M., Stute, M., Snæbjörnsdottir, S. Ó., Oelkers, E. H., Gislason, S. R., Aradottir, E. S., … & Broecker, W. S. (2016). Rapid carbon mineralization for permanent disposal of anthropogenic carbon dioxide emissions. Science, 352(6291), 1312-1314. https://doi.org/10.1126/science.aad8132
Okoko, G. O., & Olaka, L. A. (2021). Can East African rift basalts sequester CO2? Case study of the Kenya rift. Scientific African, 13, e00924. https://doi.org/10.1016/j.sciaf.2021.e00924
Pokrovsky, O. S., Golubev, S. V., Schott, J., & Castillo, A. (2009). Calcite, dolomite and magnesite dissolution kinetics in aqueous solutions at acid to circumneutral pH, 25 to 150 C and 1 to 55 atm pCO2: New constraints on CO2 sequestration in sedimentary basins. Chemical geology, 265(1-2), 20-32. https://doi.org/10.1016/j.chemgeo.2009.01.013
White, S. P., Allis, R. G., Moore, J., Chidsey, T., Morgan, C., Gwynn, W., & Adams, M. (2005). Simulation of reactive transport of injected CO2 on the Colorado Plateau, Utah, USA. Chemical geology, 217(3-4), 387-405. https://doi.org/10.1016/j.chemgeo.2004.12.020
Discover more from Future of Society
Subscribe to get the latest posts sent to your email.
Well done!